
Solar constant is the amount of solar energy incident perpendicularly per unit time on the unit surface area of the Earth at an average distance between the Sun and the Earth. It values ${{S}_{\circ }}=1340w/{{m}^{2}}$.Here calculate temperature of the Sun’s surface. Radius of the Sun ${{R}_{S}}=7\times {{10}^{8}}m$
Average distance between Earth and the Sub ${{R}_{\circ }}=1.5\times {{10}^{11}}m$
$\sigma =5.67\times {{10}^{-8}}W{{m}^{-2}}{{K}^{4}}$
Answer
496.2k+ views
Hint: In the above question we are asked to determine the temperature of the surface of the Sun. First we will determine the total power radiated by the Sun at the position where the Earth is located with respect to the sun. Further we will use Stefan’s law of Power in order to determine the temperature on the surface of the Sun.
Formula used:
$P={{S}_{\circ }}4\pi {{d}^{2}}$
$P=\sigma A{{T}^{4}}$
Complete step by step answer:
To begin with let us first determine the power radiated by the sun on the Earth. Let us say the distance between the Sun and the Earth is ${R_ \circ }$ . If the solar constant ${S_ \circ }$ is the solar constant, then the Power P is equal to,
$P = {S_ \circ }4\pi {R_ \circ }^2...(1)$
If T is the temperature of the Sun and A is its surface area, then the Power radiated by the sun is equal to by Stefan’s law the power radiated by the sun is given by,
$P = \sigma A{T^4}$
If ${R_S}$ is the radius of the Sun, then we can write the above expression as,
$P = \sigma (4\pi {R_S}^2){T^4}...(2)$
Equating equation 1 and 2 we get,
$\eqalign{
& P = {S_ \circ }4\pi {R_ \circ }^2 = \sigma (4\pi {R_S}^2){T^4} \cr
& \Rightarrow {T^4} = \dfrac{{{S_ \circ }4\pi {R_ \circ }^2}}{{\sigma 4\pi {R_S}^2}} \cr
& \Rightarrow T = {\left( {\dfrac{{{S_ \circ }{R_ \circ }^2}}{{\sigma {R_S}^2}}} \right)^{1/4}} \cr} $
Now substituting the required quantities in the above expression, the temperature of the surface of the Sun we get as,
$\eqalign{
& T = {\left( {\dfrac{{1340w/{m^2}{{(1.5 \times {{10}^{11}}m)}^2}}}{{5.67 \times {{10}^{ - 8}}W{m^{ - 2}}{K^4}{{(7 \times {{10}^8}m)}^2}}}} \right)^{1/4}} \cr
& \Rightarrow T = {\left( {\dfrac{{1340 \times 2.25 \times {{10}^{22}}}}{{5.67 \times {{10}^{ - 8}} \times 49 \times {{10}^{16}}m}}} \right)^{1/4}} \cr
& \therefore T = 5775.8{\text{ }}K \cr} $
Note: $\sigma $ is a universal constant called the Stefan-Boltzmann constant. This is basically due to the fact that the relation for power radiated by the black body was first experimentally deduced by Stefan and later proved theoretically by Boltzmann. IF in a case if a body is not a perfectly black body and has some emissivity $\in $ , then the above relation for power becomes $P=\in \sigma A{{T}^{4}}$ . It is also to be noted that energy radiated also depends on the temperature of the enclosure of the body which radiates the energy.
Formula used:
$P={{S}_{\circ }}4\pi {{d}^{2}}$
$P=\sigma A{{T}^{4}}$
Complete step by step answer:
To begin with let us first determine the power radiated by the sun on the Earth. Let us say the distance between the Sun and the Earth is ${R_ \circ }$ . If the solar constant ${S_ \circ }$ is the solar constant, then the Power P is equal to,
$P = {S_ \circ }4\pi {R_ \circ }^2...(1)$
If T is the temperature of the Sun and A is its surface area, then the Power radiated by the sun is equal to by Stefan’s law the power radiated by the sun is given by,
$P = \sigma A{T^4}$
If ${R_S}$ is the radius of the Sun, then we can write the above expression as,
$P = \sigma (4\pi {R_S}^2){T^4}...(2)$
Equating equation 1 and 2 we get,
$\eqalign{
& P = {S_ \circ }4\pi {R_ \circ }^2 = \sigma (4\pi {R_S}^2){T^4} \cr
& \Rightarrow {T^4} = \dfrac{{{S_ \circ }4\pi {R_ \circ }^2}}{{\sigma 4\pi {R_S}^2}} \cr
& \Rightarrow T = {\left( {\dfrac{{{S_ \circ }{R_ \circ }^2}}{{\sigma {R_S}^2}}} \right)^{1/4}} \cr} $
Now substituting the required quantities in the above expression, the temperature of the surface of the Sun we get as,
$\eqalign{
& T = {\left( {\dfrac{{1340w/{m^2}{{(1.5 \times {{10}^{11}}m)}^2}}}{{5.67 \times {{10}^{ - 8}}W{m^{ - 2}}{K^4}{{(7 \times {{10}^8}m)}^2}}}} \right)^{1/4}} \cr
& \Rightarrow T = {\left( {\dfrac{{1340 \times 2.25 \times {{10}^{22}}}}{{5.67 \times {{10}^{ - 8}} \times 49 \times {{10}^{16}}m}}} \right)^{1/4}} \cr
& \therefore T = 5775.8{\text{ }}K \cr} $
Note: $\sigma $ is a universal constant called the Stefan-Boltzmann constant. This is basically due to the fact that the relation for power radiated by the black body was first experimentally deduced by Stefan and later proved theoretically by Boltzmann. IF in a case if a body is not a perfectly black body and has some emissivity $\in $ , then the above relation for power becomes $P=\in \sigma A{{T}^{4}}$ . It is also to be noted that energy radiated also depends on the temperature of the enclosure of the body which radiates the energy.
Recently Updated Pages
Master Class 11 Economics: Engaging Questions & Answers for Success
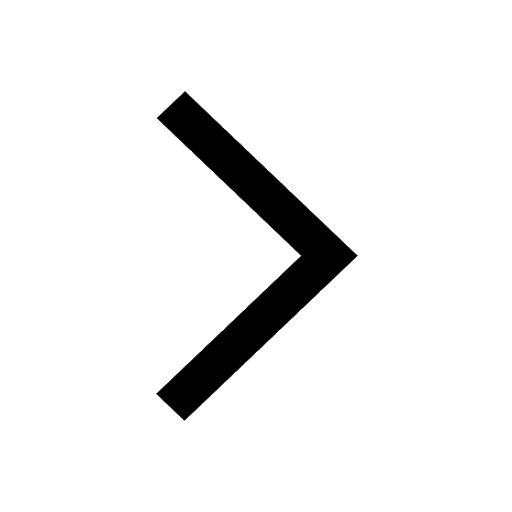
Master Class 11 Accountancy: Engaging Questions & Answers for Success
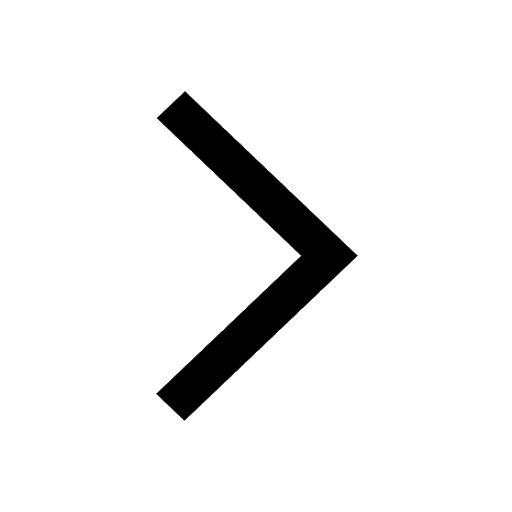
Master Class 11 English: Engaging Questions & Answers for Success
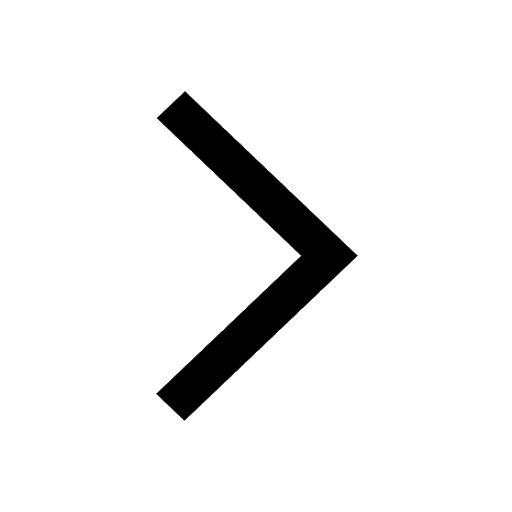
Master Class 11 Social Science: Engaging Questions & Answers for Success
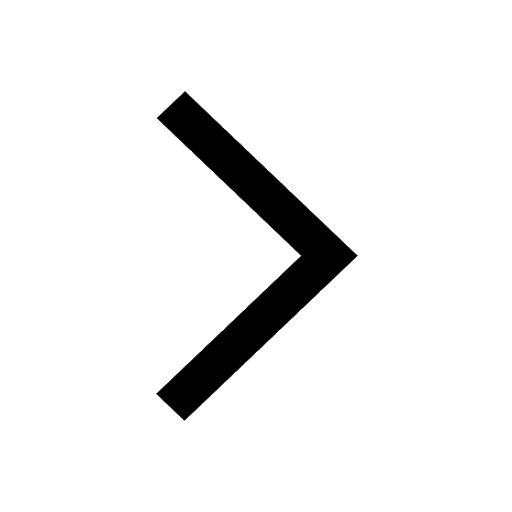
Master Class 11 Physics: Engaging Questions & Answers for Success
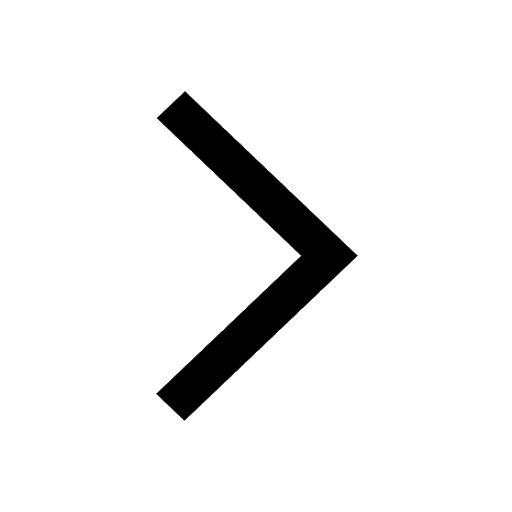
Master Class 11 Biology: Engaging Questions & Answers for Success
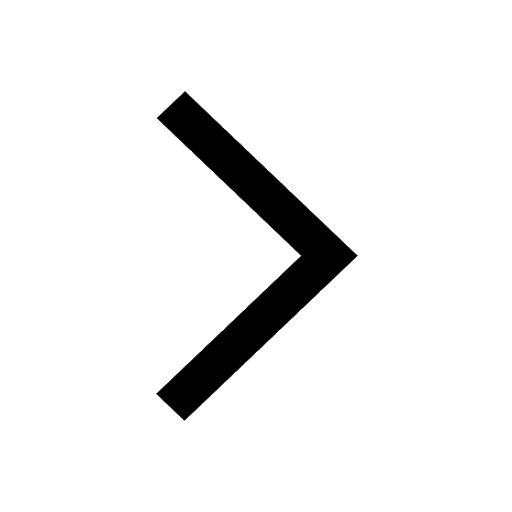
Trending doubts
1 ton equals to A 100 kg B 1000 kg C 10 kg D 10000 class 11 physics CBSE
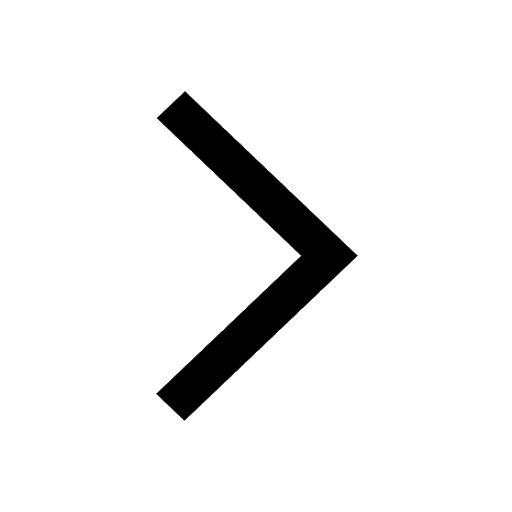
Difference Between Prokaryotic Cells and Eukaryotic Cells
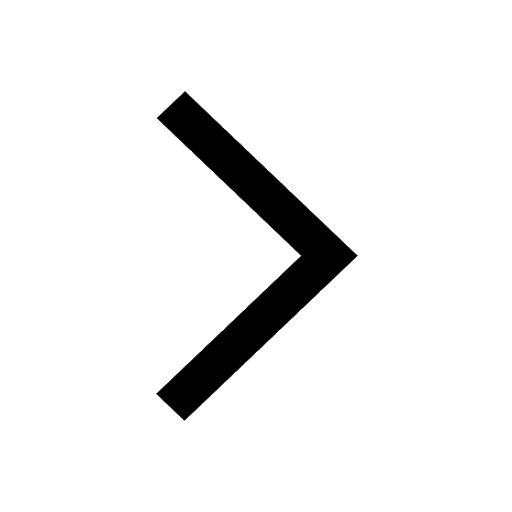
Whales are warmblooded animals which live in cold seas class 11 biology CBSE
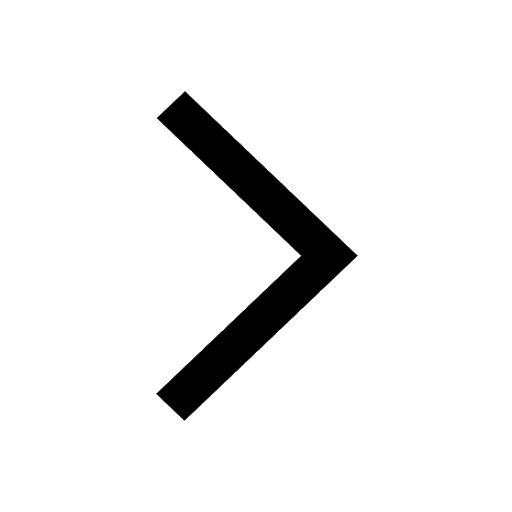
One Metric ton is equal to kg A 10000 B 1000 C 100 class 11 physics CBSE
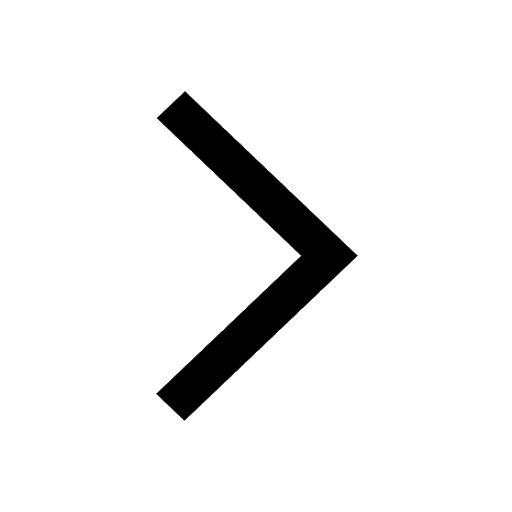
How much is 23 kg in pounds class 11 chemistry CBSE
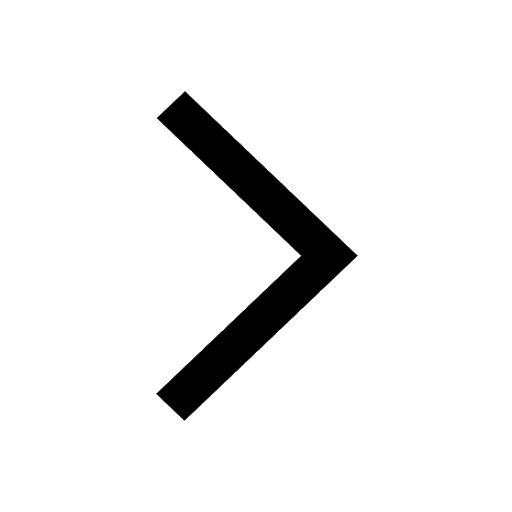
Explain zero factorial class 11 maths CBSE
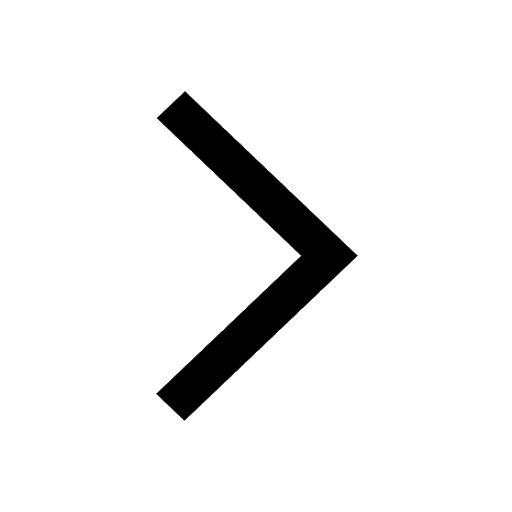