
Explain the binding energy curve. Write its importance. What is gamma decay? Explain with an example.
Answer
502.5k+ views
Hint: In this question use the concepts that binding energy curve is the plot between the average binding energy per nucleon in (Mev) and the number of nucleons in nucleus, moreover gamma rays are produced during gamma decay use this to get the definition of gamma decay and think of any reaction in which gamma ray is ejected, this will be the example for the decay.
Complete Step-by-Step solution:
Binding energy curve:
In the periodic table of elements, the series of light elements from hydrogen up to sodium is observed to exhibit generally increasing binding energy per nucleon as the atomic mass increases. This increase is generated by increasing forces per nucleon in the nucleus, as each additional nucleon is attracted by other nearby nucleons, and thus more tightly bound to the whole.
The region of increasing binding energy is followed by a region of relative stability (saturation) in the sequence from magnesium through xenon. In this region, the nucleus has become large enough that nuclear forces no longer completely extend efficiently across its width. Attractive nuclear forces in this region, as atomic mass increases, are nearly balanced by repellent electromagnetic forces between protons, as the atomic number increases.
Finally, in elements heavier than xenon, there is a decrease in binding energy per nucleon as atomic number increases. In this region of nuclear size, electromagnetic repulsive forces are beginning to overcome the strong nuclear force attraction.
Importance of binding energy:
Nuclear binding energy is the minimum energy that would be required to disassemble the nucleus of an atom into its component parts. These component parts are neutrons and protons, which are collectively called nucleons. The binding energy is always a positive number, as we need to spend energy in moving these nucleons, attracted to each other by the strong nuclear force, away from each other. The mass of an atomic nucleus is less than the sum of the individual masses of the free constituent protons and neutrons, according to Einstein's equation $E = m{c^2}$. This 'missing mass' is known as the mass defect, and represents the energy that was released when the nucleus was formed.
Gamma decay:
Gamma rays are produced during gamma decay, which normally occurs after other forms of decay occur, such as alpha or beta decay. A radioactive nucleus can decay by the emission of an $\alpha $ or $\beta $particle. The daughter nucleus that results is usually left in an excited state. It can then decay to a lower energy state by emitting a gamma ray photon, in a process called gamma decay.
And it is denoted by $\gamma $.
Example:
One example of gamma ray production due to radionuclide decay is the decay scheme for cobalt-60,
Which is shown below,
${}_{27}C{o^{60}} \to {\left( {{}_{28}N{i^{60}}} \right)^ * } + {e^ - }\left( {0.31Mev} \right) + \gamma + 1.17Mev$
${\left( {{}_{28}N{i^{60}}} \right)^ * } \to {}_{28}N{i^{60}} + \gamma + 1.33Mev$
So first cobalt (Co) decay into excited (*) nickel (Ni) by beta decay emission of an electron of 0.31 Mev, then the excited Ni decays to the ground state by emitting gamma rays in succession of 1.17 Mev followed by 1.33 Mev, this path is followed 99.88 % of time.
So this is the required answer.
Note – Gamma decay is also termed as gamma emission, gamma rays are photons or the packets of electromagnetic energy which are of extremely short wavelength. Gamma decay occurs when a nucleus is in an excited state and has too much energy to be stable, this often happens after the alpha or the beta decay has occurred. Binding energy is the energy that holds a nucleus together and it is equal to the mass defect of the nucleus.
Complete Step-by-Step solution:
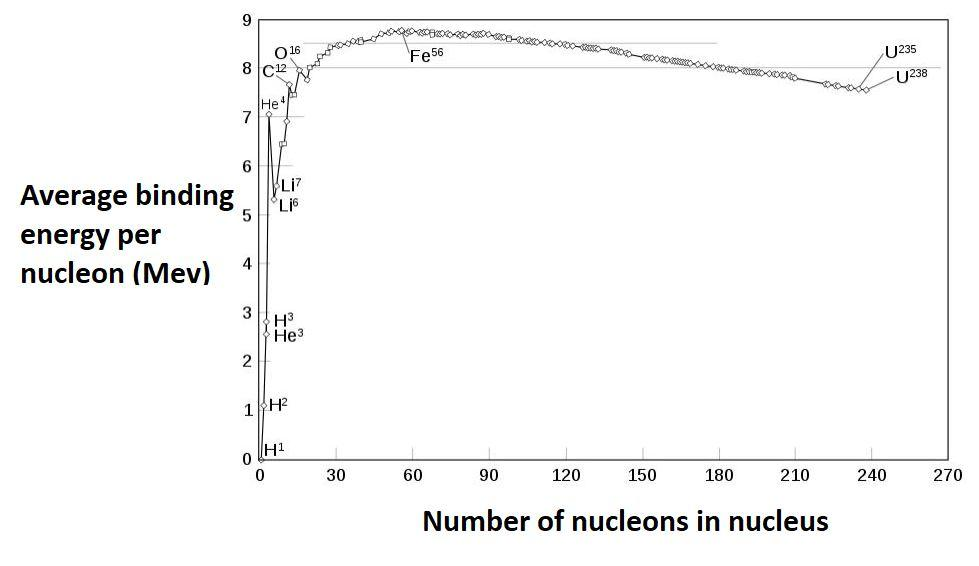
Binding energy curve:
In the periodic table of elements, the series of light elements from hydrogen up to sodium is observed to exhibit generally increasing binding energy per nucleon as the atomic mass increases. This increase is generated by increasing forces per nucleon in the nucleus, as each additional nucleon is attracted by other nearby nucleons, and thus more tightly bound to the whole.
The region of increasing binding energy is followed by a region of relative stability (saturation) in the sequence from magnesium through xenon. In this region, the nucleus has become large enough that nuclear forces no longer completely extend efficiently across its width. Attractive nuclear forces in this region, as atomic mass increases, are nearly balanced by repellent electromagnetic forces between protons, as the atomic number increases.
Finally, in elements heavier than xenon, there is a decrease in binding energy per nucleon as atomic number increases. In this region of nuclear size, electromagnetic repulsive forces are beginning to overcome the strong nuclear force attraction.
Importance of binding energy:
Nuclear binding energy is the minimum energy that would be required to disassemble the nucleus of an atom into its component parts. These component parts are neutrons and protons, which are collectively called nucleons. The binding energy is always a positive number, as we need to spend energy in moving these nucleons, attracted to each other by the strong nuclear force, away from each other. The mass of an atomic nucleus is less than the sum of the individual masses of the free constituent protons and neutrons, according to Einstein's equation $E = m{c^2}$. This 'missing mass' is known as the mass defect, and represents the energy that was released when the nucleus was formed.
Gamma decay:
Gamma rays are produced during gamma decay, which normally occurs after other forms of decay occur, such as alpha or beta decay. A radioactive nucleus can decay by the emission of an $\alpha $ or $\beta $particle. The daughter nucleus that results is usually left in an excited state. It can then decay to a lower energy state by emitting a gamma ray photon, in a process called gamma decay.
And it is denoted by $\gamma $.
Example:
One example of gamma ray production due to radionuclide decay is the decay scheme for cobalt-60,
Which is shown below,
${}_{27}C{o^{60}} \to {\left( {{}_{28}N{i^{60}}} \right)^ * } + {e^ - }\left( {0.31Mev} \right) + \gamma + 1.17Mev$
${\left( {{}_{28}N{i^{60}}} \right)^ * } \to {}_{28}N{i^{60}} + \gamma + 1.33Mev$
So first cobalt (Co) decay into excited (*) nickel (Ni) by beta decay emission of an electron of 0.31 Mev, then the excited Ni decays to the ground state by emitting gamma rays in succession of 1.17 Mev followed by 1.33 Mev, this path is followed 99.88 % of time.
So this is the required answer.
Note – Gamma decay is also termed as gamma emission, gamma rays are photons or the packets of electromagnetic energy which are of extremely short wavelength. Gamma decay occurs when a nucleus is in an excited state and has too much energy to be stable, this often happens after the alpha or the beta decay has occurred. Binding energy is the energy that holds a nucleus together and it is equal to the mass defect of the nucleus.
Recently Updated Pages
Master Class 10 Computer Science: Engaging Questions & Answers for Success
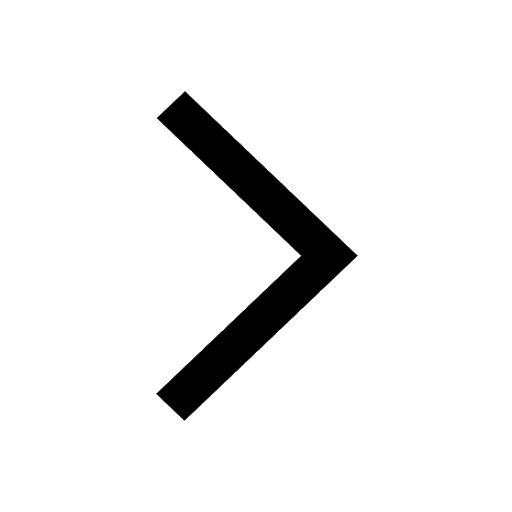
Master Class 10 Science: Engaging Questions & Answers for Success
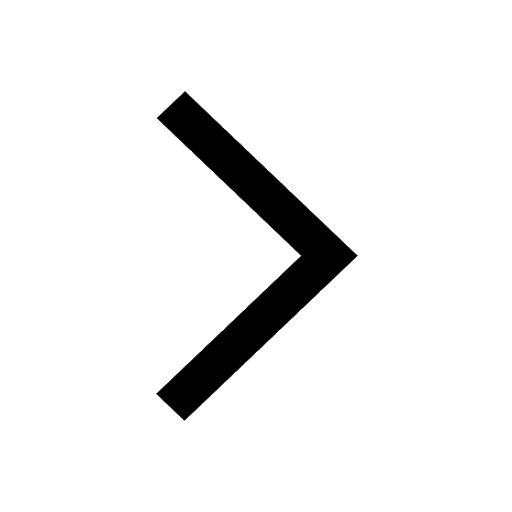
Master Class 10 Social Science: Engaging Questions & Answers for Success
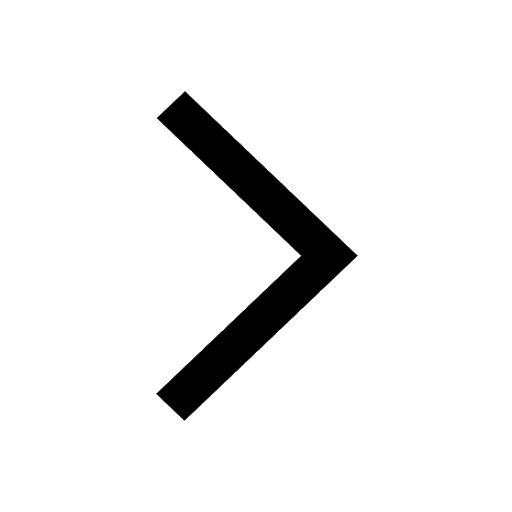
Master Class 10 Maths: Engaging Questions & Answers for Success
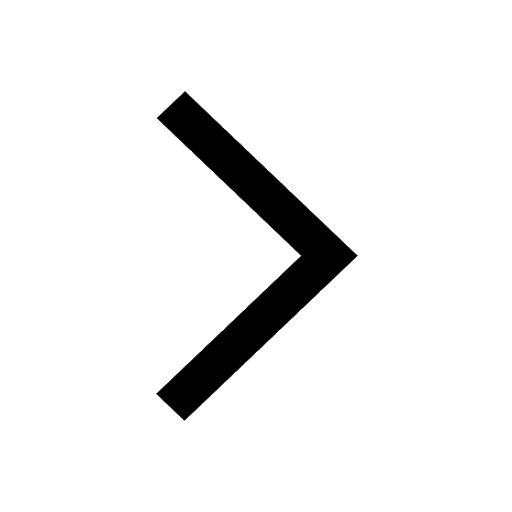
Master Class 10 English: Engaging Questions & Answers for Success
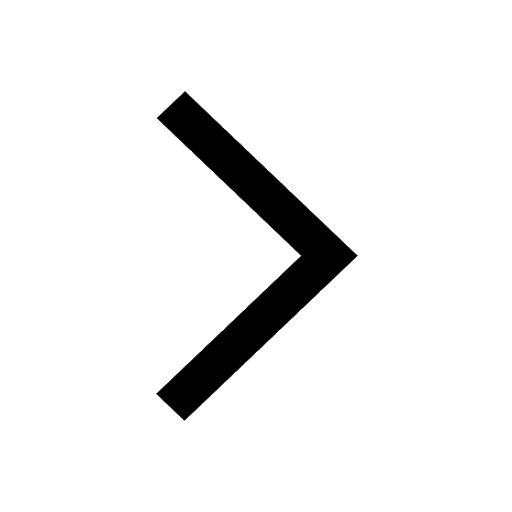
Class 10 Question and Answer - Your Ultimate Solutions Guide
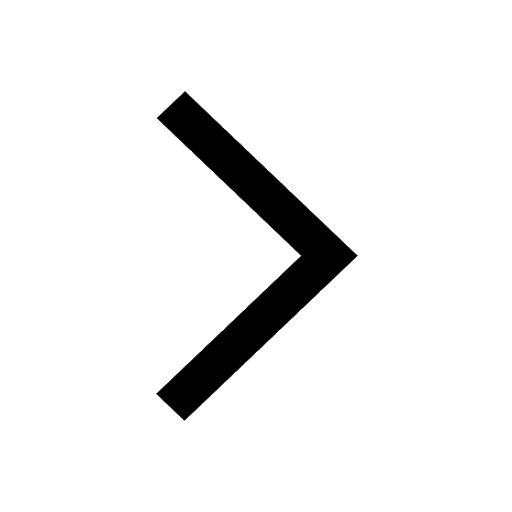
Trending doubts
The Equation xxx + 2 is Satisfied when x is Equal to Class 10 Maths
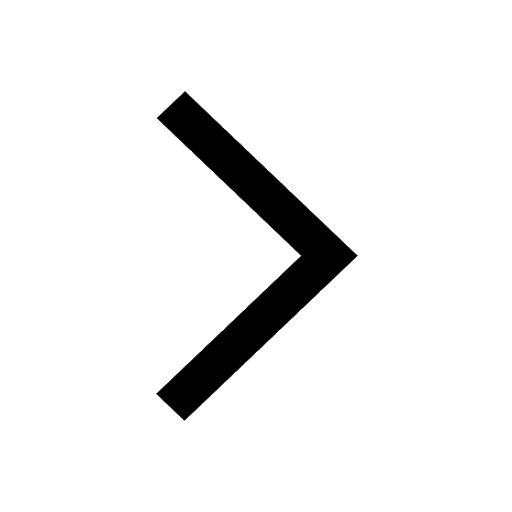
Why is there a time difference of about 5 hours between class 10 social science CBSE
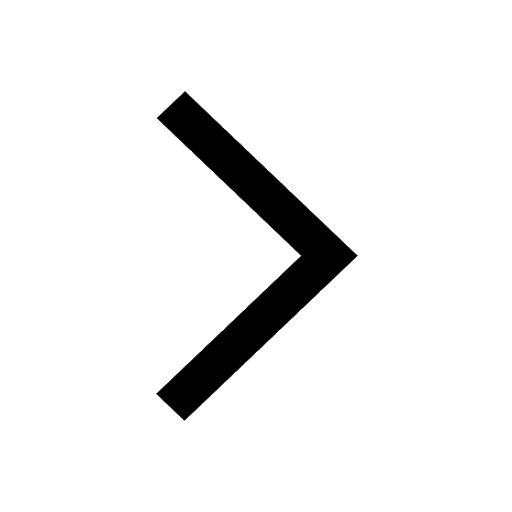
Change the following sentences into negative and interrogative class 10 english CBSE
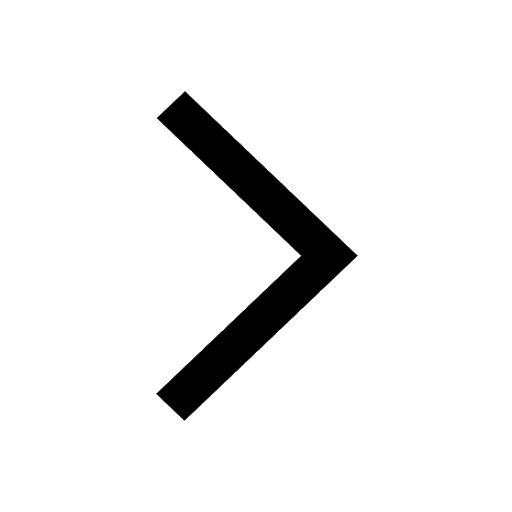
What constitutes the central nervous system How are class 10 biology CBSE
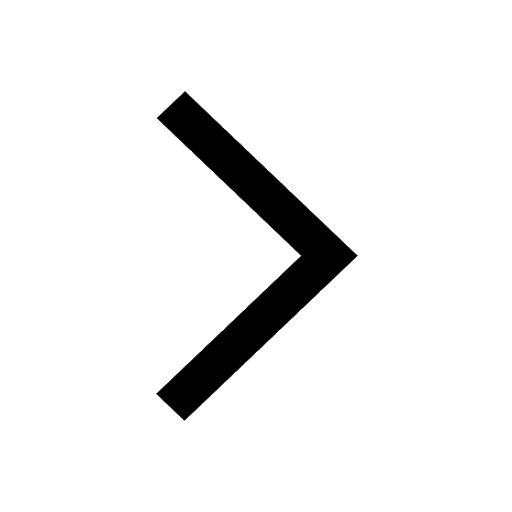
Write a letter to the principal requesting him to grant class 10 english CBSE
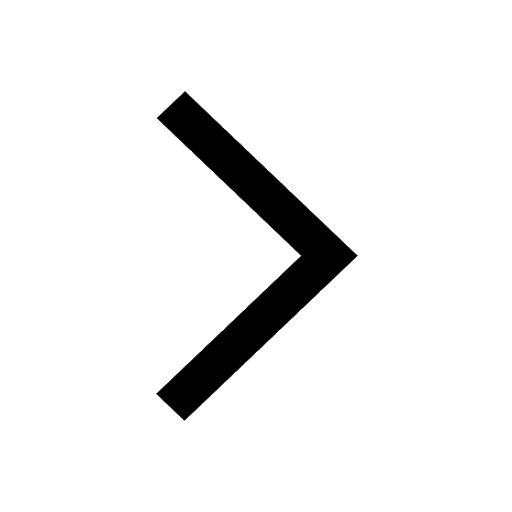
Explain the Treaty of Vienna of 1815 class 10 social science CBSE
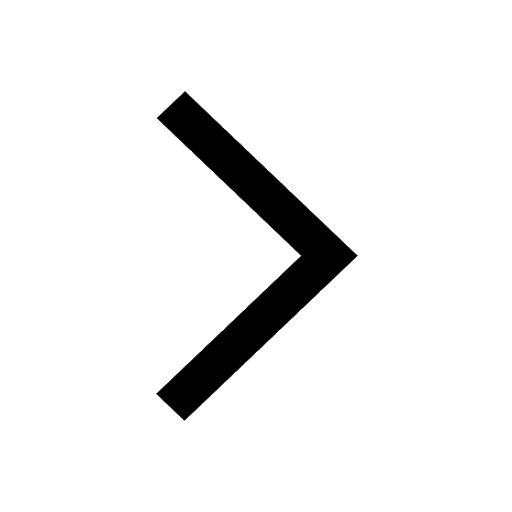